Volume 6, Number 5—October 2000
Synopsis
Antigenic Variation in Vector-Borne Pathogens
Figure 1
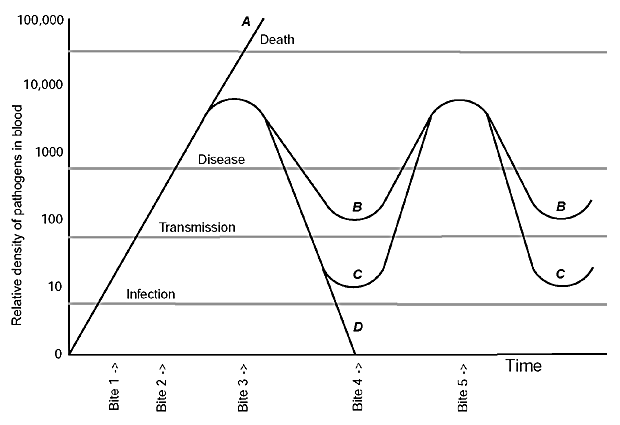
Figure 1. Relative densities of a vector-borne pathogen in the blood of four hosts, A-D. The gray horizontal lines represent the lower thresholds for the persistence of infection, transmission to a new vector, symptomatic disease in the host, and death of the host. In host A, overwhelming infection causes death. In hosts B and C, there is antigenic variation of the pathogen; B differs from C in the continuing likelihood of transmission even during periods of no or little illness. In case C, the host remains infected but is not infectious between relapses of high-density parasitemia and illness. In host D, the pathogen is cleared from the blood by the immune system. The relative density of the pathogens in the blood is on the y axis. If the arthropod vector of the infection fed on the four hosts at different times, the following outcomes would be observed: a bite at time 1 would not result in transmission because the density of pathogens in the blood was insufficient for uptake and establishment of the pathogen in the vector. At times 2 or 3, the disease agent is transmitted to the biting arthropod, although the hosts bitten at time 2 are not yet ill. At time 3, the infection worsens, in case A in the absence of an effective immune response. In cases B-D, the infection peaks as neutralizing antibodies to the infecting serotype appear in the blood at time 3. An arthropod taking a blood meal at time 4 could acquire the infection from host B but not from hosts C or D. In case D, the infection has been cleared by time 4. At time 5, both B and C could transmit the pathogens again and both have a relapse of illness as the result of the proliferation of a new serotype. The figure is modified from a figure by Turner (7).
References
- Wang IN, Dykhuizen DE, Qiu W, Dunn JJ, Bosler EM, Luft BJ. Genetic diversity of ospC in a local population of Borrelia burgdorferi sensu stricto. Genetics. 1999;151:15–30.PubMedGoogle Scholar
- Stevenson B, Bockenstedt LK, Barthold SW. Expression and gene sequence of outer surface protein C of Borrelia burgdorferi reisolated from chronically infected mice. Infect Immun. 1994;62:3568–71.PubMedGoogle Scholar
- Beale GH. J.F. W. Antigenic variation in unicellular organisms. Annu Rev Microbiol. 1961;15:263–96. DOIGoogle Scholar
- Barbour AG, Hayes SF. Biology of Borrelia species. Microbiol Rev. 1986;50:381–400.PubMedGoogle Scholar
- de la Vega I, Gonzalez A, Blasco R, Calvo V, Vinuela E. Nucleotide sequence and variability of the inverted terminal repetitions of African swine fever virus DNA. Virology. 1994;201:152–6. DOIPubMedGoogle Scholar
- Zuckert WR, Meyer J, Barbour AG. Comparative analysis and immunological characterization of the Borrelia Bdr protein family. Infect Immun. 1999;67:3257–66.PubMedGoogle Scholar
- Turner CM. Antigenic variation in Trypanosoma brucei infections: an holistic view. J Cell Sci. 1999;112:3187–92.PubMedGoogle Scholar
- Barbour AG. Immunobiology of relapsing fever. Contrib Microbiol Immunol. 1987;8:125–37.PubMedGoogle Scholar
- Vickerman K. Trypanosome sociology and antigenic variation. Parasitology. 1989;99:S37–47. DOIPubMedGoogle Scholar
- Deitsch KW, Moxon ER, Wellems TE. Shared themes of antigenic variation and virulence in bacterial, protozoal, and fungal infections. Microbiol Mol Biol Rev. 1997;61:281–93.PubMedGoogle Scholar
- Hinnebusch BJ, Barbour AG, Restrepo BI, Schwan TG. Population structure of the relapsing fever spirochete Borrelia hermsii as indicated by polymorphism of two multigene families that encode immunogenic outer surface lipoproteins. Infect Immun. 1998;66:432–40.PubMedGoogle Scholar
- Barbour AG. Linear DNA of Borrelia species and antigenic variation. Trends Microbiol. 1993;1:236–9. DOIPubMedGoogle Scholar
- Stoenner HG, Dodd T, Larsen C. Antigenic variation of Borrelia hermsii. J Exp Med. 1982;156:1297–311. DOIPubMedGoogle Scholar
- Barbour AG, Tessier SL, Stoenner HG. Variable major proteins of Borrellia hermsii. J Exp Med. 1982;156:1312–24. DOIPubMedGoogle Scholar
- Burman N, Bergstrom S, Restrepo BI, Barbour AG. The variable antigens Vmp7 and Vmp21 of the relapsing fever bacterium Borrelia hermsii are structurally analogous to the VSG proteins of the African trypanosome. Mol Microbiol. 1990;4:1715–26. DOIPubMedGoogle Scholar
- Restrepo BI, Kitten T, Carter CJ, Infante D, Barbour AG. Subtelomeric expression regions of Borrelia hermsii linear plasmids are highly polymorphic. Mol Microbiol. 1992;6:3299–311. DOIPubMedGoogle Scholar
- Vidal V, Scragg IG, Cutler SJ, Rockett KA, Fekade D, Warrell DA, Variable major lipoprotein is a principal TNF-inducing factor of louse-borne relapsing fever. Nat Med. 1998;4:1416–20. DOIPubMedGoogle Scholar
- Shamaei-Tousi A, Martin P, Bergh A, Burman N, Brännström T, Bergström S. Erythrocyte-aggregating relapsing fever spirochete Borrelia crocidurae induces formation of microemboli. J Infect Dis. 1999;180:1929–38. DOIPubMedGoogle Scholar
- Pennington PM, Cadavid D, Bunikis J, Norris SJ, Barbour AG. Extensive interplasmidic duplications change the virulence phenotype of the relapsing fever agent Borrelia turicatae. Mol Microbiol. 1999;34:1120–32. DOIPubMedGoogle Scholar
- Cadavid D, Thomas DD, Crawley R, Barbour AG. Variability of a bacterial surface protein and disease expression in a possible mouse model of systemic Lyme borreliosis. J Exp Med. 1994;179:631–42. DOIPubMedGoogle Scholar
- Pennington PM, Allred CD, West CS, Alvarez R, Barbour AG. Arthritis severity and spirochete burden are determined by serotype in the Borrelia turicatae-mouse model of Lyme disease. Infect Immun. 1997;65:285–92.PubMedGoogle Scholar
- Kitten T, Barbour AG. Juxtaposition of expressed variable antigen genes with a conserved telomere in the bacterium Borrelia hermsii. Proc Natl Acad Sci U S A. 1990;87:6077–81. DOIPubMedGoogle Scholar
- Barbour AG, Burman N, Carter CJ, Kitten T, Bergstrom S. Variable antigen genes of the relapsing fever agent Borrelia hermsii are activated by promoter addition. Mol Microbiol. 1991;5:489–93. DOIPubMedGoogle Scholar
- Kitten T, Barrera AV, Barbour AG. Intragenic recombination and a chimeric outer membrane protein in the relapsing fever agent Borrelia hermsii. J Bacteriol. 1993;175:2516–22.PubMedGoogle Scholar
- Restrepo BI, Carter CJ, Barbour AG. Activation of a vmp pseudogene in Borrelia hermsii: an alternate mechanism of antigenic variation during relapsing fever. Mol Microbiol. 1994;13:287–99. DOIPubMedGoogle Scholar
- Restrepo BI, Barbour AG. Antigen diversity in the bacterium B. hermsii through "somatic" mutations in rearranged vmp genes. Cell. 1994;78:867–76. DOIPubMedGoogle Scholar
- Carter CJ, Bergstrom S, Norris SJ, Barbour AG. A family of surface-exposed proteins of 20 kilodaltons in the genus Borrelia. Infect Immun. 1994;62:2792–9.PubMedGoogle Scholar
- Barbour AG, Carter CJ, Sohaskey CD. Surface protein variation by expression site switching in the relapsing fever agent Borrelia hermsii. Infect Immun. 2000. In press.
- Schwan TG, Hinnebusch BJ. Bloodstream- versus tick-associated variants of a relapsing fever bacterium. Science. 1998;280:1938–40. DOIPubMedGoogle Scholar
- Zhang JR, Hardham JM, Barbour AG, Norris SJ. Antigenic variation in Lyme disease borreliae by promiscuous recombination of VMP-like sequence cassettes. Cell. 1997;89:275–85. DOIPubMedGoogle Scholar
- Zhang JR, Norris SJ. Kinetics and in vivo induction of genetic variation of vlsE in Borrelia burgdorferi. Infect Immun. 1998;66:3689–97.PubMedGoogle Scholar
- Kieser ST, Eriks IS, Palmer GH. Cyclic rickettsemia during persistent Anaplasma marginale infection of cattle. Infect Immun. 1990;58:1117–9.PubMedGoogle Scholar
- Palmer GH, Abbott JR, French DM, McElwain TF. Persistence of Anaplasma ovis infection and conservation of the msp-2 and msp-3 multigene families within the genus Anaplasma. Infect Immun. 1998;66:6035–9.PubMedGoogle Scholar
- Sulsona CR, Mahan SM, Barbet AF. The map1 gene of Cowdria ruminantium is a member of a multigene family containing both conserved and variable genes. Biochem Biophys Res Commun. 1999;257:300–5. DOIPubMedGoogle Scholar
- Zhi N, Ohashi N, Rikihisa Y. Multiple p44 genes encoding major outer membrane proteins are expressed in the human granulocytic ehrlichiosis agent. J Biol Chem. 1999;274:17828–36. DOIPubMedGoogle Scholar
- French DM, Brown WC, Palmer GH. Emergence of Anaplasma marginale antigenic variants during persistent rickettsemia. Infect Immun. 1999;67:5834–40.PubMedGoogle Scholar
- Rudenko G, Cross M, Borst P. Changing the end: antigenic variation orchestrated at the telomeres of African trypanosomes. Trends Microbiol. 1998;6:113–6. DOIPubMedGoogle Scholar
- Lamont GS, Tucker RS, Cross GA. Analysis of antigen switching rates in Trypanosoma brucei. Parasitology. 1986;92:355–67. DOIPubMedGoogle Scholar
- Turner CM. The rate of antigenic variation in fly-transmitted and syringe-passaged infections of Trypanosoma brucei. FEMS Microbiol Lett. 1997;153:227–31. DOIPubMedGoogle Scholar
- Pays E, Tebabi P, Pays A, Coquelet H, Revelard P, Salmon D, The genes and transcripts of an antigen gene expression site from T. brucei. Cell. 1989;57:835–45. DOIPubMedGoogle Scholar
- Lips S, Revelard P, Pays E. Identification of a new expression site-associated gene in the complete 30.5 kb sequence from the AnTat 1.3A variant surface protein gene expression site of Trypanosoma brucei. Mol Biochem Parasitol. 1993;62:135–7. DOIPubMedGoogle Scholar
- Bitter W, Gerrits H, Kieft R, Borst P. The role of transferrin-receptor variation in the host range of Trypanosoma brucei. Nature. 1998;391:499–502. DOIPubMedGoogle Scholar
- Baltz T, Giroud C, Bringaud F, Eisen H, Jacquemot C, Roth CW. Exposed epitopes on a Trypanosoma equiperdum variant surface glycoprotein altered by point mutations. EMBO J. 1991;10:1653–9.PubMedGoogle Scholar
- Lu Y, Alarcon CM, Hall T, Reddy LV, Donelson JE. A strand bias occurs in point mutations associated with variant surface glycoprotein gene conversion in Trypanosoma rhodesiense. Mol Cell Biol. 1994;14:3971–80.PubMedGoogle Scholar
- Gommers-Ampt J, Lutgerink J, Borst P. A novel DNA nucleotide in Trypanosoma brucei only present in the mammalian phase of the life-cycle. Nucleic Acids Res. 1991;19:1745–51. DOIPubMedGoogle Scholar
- McCulloch R, Barry JD. A role for RAD51 and homologous recombination in Trypanosoma brucei antigenic variation. Genes Dev. 1999;13:2875–88. DOIPubMedGoogle Scholar
- Rudenko G, Blundell PA, Dirks-Mulder A, Kieft R, Borst P. A ribosomal DNA promoter replacing the promoter of a telomeric VSG gene expression site can be efficiently switched on and off in T. brucei. Cell. 1995;83:547–53. DOIPubMedGoogle Scholar
- Roberts DJ, Craig AG, Berendt AR, Pinches R, Nash G, Marsh K, Rapid switching to multiple antigenic and adhesive phenotypes in malaria. Nature. 1992;357:689–92. DOIPubMedGoogle Scholar
- Baruch DI, Pasloske BL, Singh HB, Bi X, Ma XC, Feldman M, Cloning the P. falciparum gene encoding PfEMP1, a malarial variant antigen and adherence receptor on the surface of parasitized human erythrocytes. Cell. 1995;82:77–87. DOIPubMedGoogle Scholar
- Gardner JP, Pinches RA, Roberts DJ, Newbold CI. Variant antigens and endothelial receptor adhesion in Plasmodium falciparum. Proc Natl Acad Sci U S A. 1996;93:3503–8. DOIPubMedGoogle Scholar
- Smith JD, Chitnis CE, Craig AG, Roberts DJ, Hudson-Taylor DE, Peterson DS, Switches in expression of Plasmodium falciparum var genes correlate with changes in antigenic and cytoadherent phenotypes of infected erythrocytes. Cell. 1995;82:101–10. DOIPubMedGoogle Scholar
- Su XZ, Heatwole VM, Wertheimer SP, Guinet F, Herrfeldt JA, Peterson DS, The large diverse gene family var encodes proteins involved in cytoadherence and antigenic variation of Plasmodium falciparum-infected erythrocytes. Cell. 1995;82:89–100. DOIPubMedGoogle Scholar
- Hernandez-Rivas R, Mattei D, Sterkers Y, Peterson DS, Wellems TE, Scherf A. Expressed var genes are found in Plasmodium falciparum subtelomeric regions. Mol Cell Biol. 1997;17:604–11.PubMedGoogle Scholar
- Chen Q, Fernandez V, Sundstrom A, Schlichtherle M, Datta S, Hagblom P, Developmental selection of var gene expression in Plasmodium falciparum. Nature. 1998;394:392–5. DOIPubMedGoogle Scholar
- Scherf A, Hernandez-Rivas R, Buffet P, Bottius E, Benatar C, Pouvelle B, Antigenic variation in malaria: in situ switching, relaxed and mutually exclusive transcription of var genes during intra-erythrocytic development in Plasmodium falciparum. EMBO J. 1998;17:5418–26. DOIPubMedGoogle Scholar
- Kyes SA, Rowe JA, Kriek N, Newbold CI. Rifins: a second family of clonally variant proteins expressed on the surface of red cells infected with Plasmodium falciparum. Proc Natl Acad Sci U S A. 1999;96:9333–8. DOIPubMedGoogle Scholar
- Fernandez V, Hommel M, Chen Q, Hagblom P, Wahlgren M. Small, clonally variant antigens expressed on the surface of the Plasmodium falciparum-infected erythrocyte are encoded by the rif gene family and are the target of human immune responses. J Exp Med. 1999;190:1393–404. DOIPubMedGoogle Scholar
- Allred DR, Cinque RM, Lane TJ, Ahrens KP. Antigenic variation of parasite-derived antigens on the surface of Babesia bovis-infected erythrocytes. Infect Immun. 1994;62:91–8.PubMedGoogle Scholar
- O'Connor RM, Lane TJ, Stroup SE, Allred DR. Characterization of a variant erythrocyte surface antigen (VESA1) expressed by Babesia bovis during antigenic variation. Mol Biochem Parasitol. 1997;89:259–70. DOIPubMedGoogle Scholar
- O'Connor RM, Allred DR. Selection of Babesia bovis-infected erythrocytes for adhesion to endothelial cells coselects for altered variant erythrocyte surface antigen isoforms. J Immunol. 2000;164:2037–45.PubMedGoogle Scholar
- Allred DR, Carlton JM, Satcher RL, Long JA, Brown WC, Patterson PE, The ves multigene family of B. bovis encodes components of rapid antigenic variation at the infected erythrocyte surface. Mol Cell. 2000;5:153–62. DOIPubMedGoogle Scholar
- Barbour AG, Tessier SL, Todd WJ. Lyme disease spirochetes and ixodid tick spirochetes share a common surface antigenic determinant defined by a monoclonal antibody. Infect Immun. 1983;41:795–804.PubMedGoogle Scholar
- Schwan TG, Piesman J. Temporal changes in outer surface proteins A and C of the Lyme disease-associated spirochete, Borrelia burgdorferi, during the chain of infection in ticks and mice. J Clin Microbiol. 2000;38:382–8.PubMedGoogle Scholar
- Jonsson M, Noppa L, Barbour AG, Bergstrom S. Heterogeneity of outer membrane proteins in Borrelia burgdorferi: comparison of osp operons of three isolates of different geographic origins. Infect Immun. 1992;60:1845–53.PubMedGoogle Scholar
- de Silva AM, Zeidner NS, Zhang Y, Dolan MC, Piesman J, Fikrig E. Influence of outer surface protein A antibody on Borrelia burgdorferi within feeding ticks. Infect Immun. 1999;67:30–5.PubMedGoogle Scholar